Physicist: We imagine interplanetary spacecraft as massive, expensive rockets, but the Moon changes that. Spacecraft built and launched from the Moon don’t have to have huge boosters; even tiny spacecraft can travel across the solar system efficiently. We should go to the Moon because, if and when humanity expands into space, the Moon will be a major hub of transportation and industry for our Home World. It turns out that the biggest difficulty with spaceflight isn’t space, it’s Earth. And the Moon is notably not Earth.
That said, the pictures you’ve seen of the surface of the Moon are about right. It’s a terrible place. The Moon is the Ft. Lauderdale Airport of space; you only go there so you can go somewhere else.
Our Moon (the Moon) is ludicrously big relative to the Earth. The other moons in the solar system are tiny compared to their host planet and likely formed in place or were captured. The leading theory for the formation of our Moon is the “giant impact hypothesis”: another planet hit the Earth (more accurately two planets hit each other, neither/both of which were the Earth) around 4.5 billion years ago and “splashed” a huge amount of material into orbit which coalesced into the Moon. We suspect that this is the case, in part, because the samples brought back show that the surface is made of roughly the same stuff as the Earth and was once entirely molten. That’s about what you’d expect from slamming a couple planets together: space lava. The lightest of that lava was the first to solidify into a surface, leaving anorthosite for us to find. So for the most part, the stuff we expect to find (and have found) on the Moon can also be found here. The big difference isn’t material, it’s history.
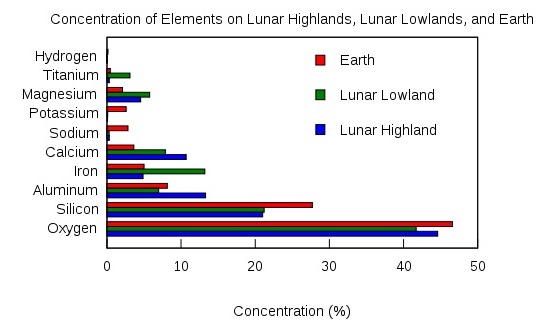
The lowlands are the dark regions, which are younger (a mere 3.2-4.2 billion years old) and formed by volcanoes. The highlands are older and formed when the Moon first cooled. And Earth is where you live (for now!).
Without a magnetic field to deflect solar wind and cosmic rays, or an atmosphere to burn up meteors, the surface of the Moon has spent the last several billion years getting pulped and nuked. Other than outcrops of bedrock poking through the surface, the entire Moon is covered in a layer of fine regolith several meters deep. This layer is the result of being melted and smashed by rocks from pebbles to mountains traveling around mach 60 (~20 km/s plus or minus a lot), all the while being irradiated by direct exposure to space and the Sun. Lunar regolith is basically an endless sea of dust and ground glass. It’s so fine that it both floats and sticks to everything electrostatically, so sharp that it damages everything it touches, and so omnipresent that there’s no escape from it. There are some clever uses for it, like using 3D printers to turn it into Moon-mud houses, but most of the time it’s just across-the-board-awful.
Eugene Cernan (Apollo 17) reported that “… one of the most aggravating, restricting facets of lunar surface exploration is the dust and its adherence to everything no matter what kind of material, whether it be skin, suit material, metal, no matter what it be and it’s restrictive friction-like action to everything it gets on” and Alan Bean (Apollo 12) kvetched that “… dust tends to rub deeper into the garment than to brush off”.
If you went to live on the Moon, the dust is the first thing you’d notice. That said, the dust and radiation are manageable. For the dust you just need a hygiene protocol to keep dust and people as separate as possible. This wasn’t really an option for the Apollo missions, which had to work from one small room with no airlock. When the world outside your front door is a nightmare, sleeping in the mud room is not ideal.
To deal with a surface that’s continually pelted with radiation and space bullets, the solution is simple: don’t be on the surface. With a dozen meters of rock between you and the sky, a lot of things get easier. No radiation, no meteors, and no extreme temperature swings (-173°C to 127°C). Luckily, we won’t even have to dig. There are hundreds of holes opening into massive ex-lava tubes running under the lunar surface. These tubes are protected from the sky, while maintaining the average lunar temperature of -21°C. Earth would have the same temperature (since we’re the same distance from the Sun), except that we’ve got an atmospheric greenhouse effect working for us (as if you needed yet another reason to be pro-air).
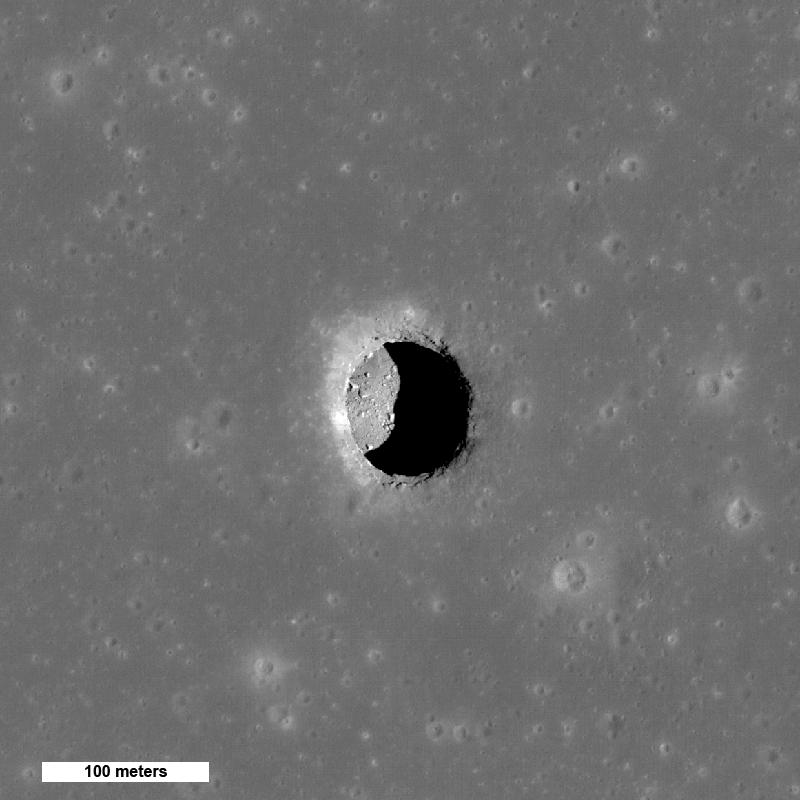
A “skylight” in the roof of a lava tube under Mare Tranquillitatis. Some of these tubes have been determined to be several hundred meters across. We can literally build cities in these things (with very simple subway systems).
That -21°C is a lot warmer than it sounds. Without air it’s difficult to get rid of heat, so cold isn’t really a practical issue in space. To experience the difference between the efficiencies of radiating heat vs. conducting/convecting heat, first sit next to a fire, then sit above it.
So the Moon is (unlikely) to be harboring mountains of gold. It’s Earth-stuff piled up in a remarkably unpleasant environment. But the Moon does have a few big selling point: it exists, it’s high up yet close by, and it’s not Earth.
Launching material from the surface of the Earth is really, really hard. Between Earth’s gravity and air, we live in one of the worst positions in the solar system for spaceflight. There’s a nigh-impenetrable wall between Earth and low orbit, an 8 km/s speed requirement, which is why launch vehicles look more like skyscrapers than spaceships. But once you’re up there, moving from place to place in space is comparatively easy.
Being small means that it takes relatively little energy to escape the Moon’s gravity and being so far from Earth means that Earth’s gravity can practically be ignored. To escape from Earth’s gravity from the surface your rocket needs to “pay a cost”: acceleration up to 11.2 km/s. To escape from as high as the Moon’s orbit, you only need to pay about 0.5 km/s toward fighting Earth’s gravity.
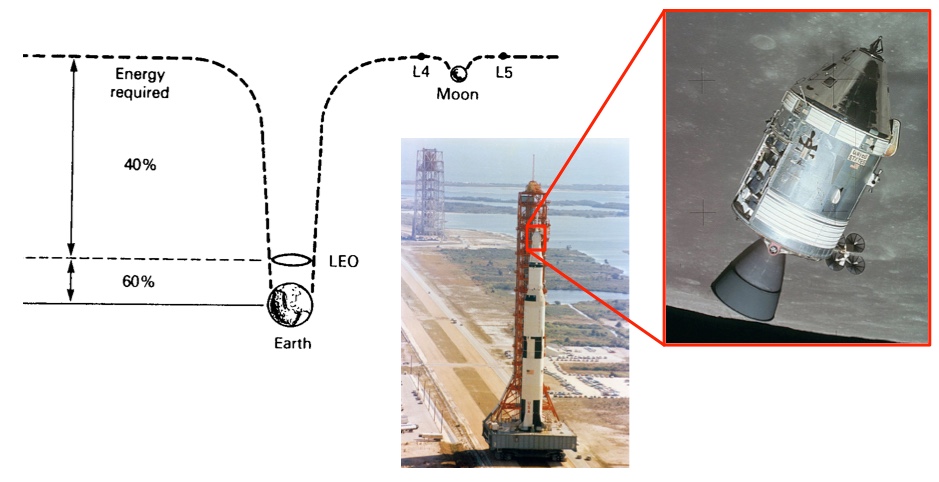
The Moon sits almost all the way out of the Earth’s gravitational potential well (left). The Saturn V (middle) was needed to launch the Command Module and LEM to lunar orbit, but the tiny Command Module (right) was all that was needed to come back. To get a sense of scale, the Saturn V rocket is about the size of Statue of Liberty and the Command Module is about the size of a truck (like those in the background of the Saturn V image).
It’s hard to emphasize how much of a head start launching from the Moon gives us. Had they been so inclined, the Apollo astronauts could have gone awol and flown the Command Module to Mars instead of returning home. Their humble spacecraft was capable of accelerating as much as 2.8 km/s and to get to Mars from lunar orbit only requires about 2.2 km/s. They would have run out of air long before they arrived and they wouldn’t have had the fuel to slow down, but the point is that they had the option to be the handsomest corpses to ever impact the Martian surface (an honor they bravely passed up).
So to build actual spacecraft and cities we have to do it off-world. There just isn’t another option. Building colossal rockets to put itty-bitty payloads into space is silly, so it’s our good fortune that the Moon exists. There’s all the makings of spaceships, habitats, fuel, and even food up there; it’s just a matter of processing it.
Earth’s 23.5° tilt means that every point on Earth will be exposed to the Sun at some point during the year. But the Moon has a far more modest 1.5° tilt, which means that the bottom of craters near the poles never see the Sun. These Craters of Eternal Darkness (that is seriously the name) are among the coldest places in the solar system, -247°C, a balmy 26°C above absolute zero. Without sunlight, these craters are able to sustain water ice that isn’t found elsewhere. It’s estimated that there is on the order of a billion tons of water, about a cubic km, of ice buried in those polar craters, which is great news. Water means easily accessible hydrogen for fuel, oxygen for breathing, water for drinking, and ice cubes for space whiskey. There are already plans in the works to start exploiting this resource, so that the Moon can be a fuel depot for trips farther out into the solar system.
Fortunately, the Craters of Eternal Darkness are rimmed by Peaks of Eternal Light (I am not making this up), which always see the Sun on the horizon. That means that unlike everywhere else on the Moon, a base at the poles never has to go without sunlight (and solar power), unlike the rest of the Moon which has two weeks of light and two weeks of night every lunar month.
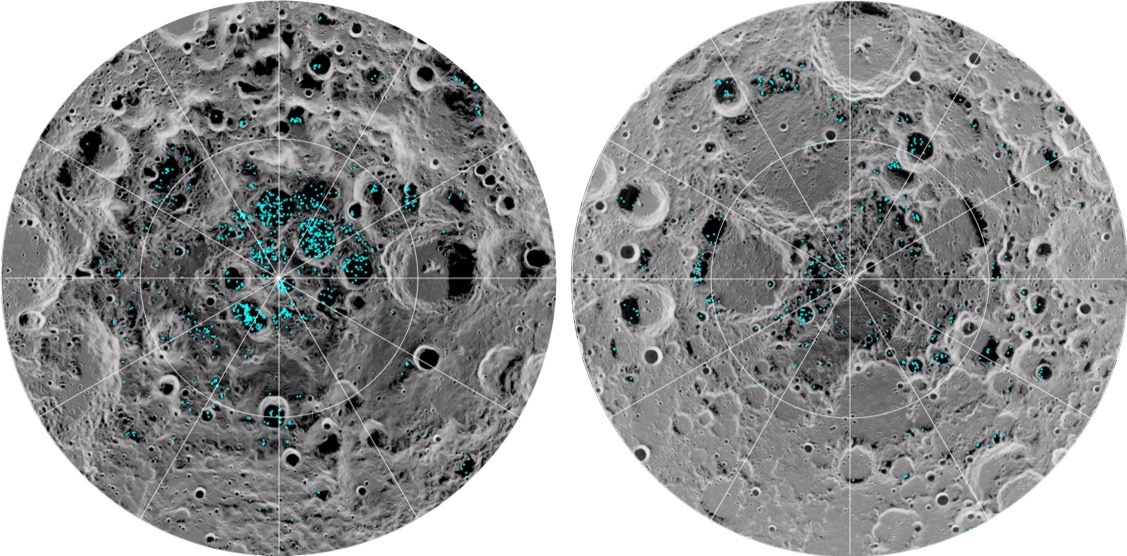
Maps of the water ice detected around the south (left) and north (right) poles of the Moon. These maps cover 10° of latitude, which is an area about the size of Italy.
This ice seems to be fantastically old and was likely deposited by comets. Not to get too far ahead of ourselves, but that means that lunar ice is a non-renewable resource. A billion tons sounds like a lot, until you consider that we’ve dug up and burned over 20 billion tons of oil in the last century and a half here on Earth. I’m sure it won’t be an issue.
But just in case it is, there are more elegant ways to get to and from the lunar surface: by rail. One of the big advantages of not having an atmosphere is not having a speed limit. To get from the surface of the Moon to the top of Earth’s atmosphere requires about 5.9 km/s. In Earth’s atmosphere 5.9 km/s is thoroughly fatal, but on the Moon the only way to notice that kind of speed is to look out a window.
A “mass driver” is basically a maglev train. Magnets support and propel the spacecraft along a rail until it reaches the desired speed. For an acceleration of 3 g’s (what you experience in a Gravitron ride at the fair) you’d need a rail 592 km long and passengers would only have to be uncomfortable for a little over three minutes. For cargo that definitely doesn’t need to survive the journey, you can ramp up the acceleration and shorten the rail by the same factor.
So why go to the Moon? It’s the first, most important step in expanding into the universe and eventually eliminating humanity’s industrial impact on Earth. The Moon is a platform that we can use to bring ourselves and our ambitions into the heavens.
Also science. But of course, it’s much easier to learn about a place when you live there.
To imagine that an accidental impact was so precise and orchestrated that it could make the moon and mak it perfectly where it could keep time and be ON TIME is ludicrous and rediculas. Accidents do not make things as perfect as our moon or our fun! Please Try again !
Another nice thing about the Moon is that it is sufficiently massive to have strong enough gravity that things will generally stay where you put them and not go floating off somewhere inconvenient, but still weak enough that leaving the Moon and going elsewhere does not require a considerable amount of energy.
And of course, if we start running out of ice on the Moon, we could always crash a comet or two into it to replenish the supply: Things crash into the Moon all the time and no-one gives a damn and there’s no atmosphere to burn it up before it reaches the ground, unlike the Earth.
@Neruz
I’ll bet Velcro™ starts to sound like fingernails on a chalkboard after you’ve been living on the ISS a while.
I am aware of the sensitivity of gravity. The moon orbits the earth in an evolved state. If it slowed down marginally, it would get closer to earth and the force of gravity would increase noticeably. The people of NASA are of course aware of this, but I have never heard it discussed openly. I am also aware of quantum mechanics stability for electron states. Perhaps it all adjusts itself slowly. I just hope we are thinking about the balance and watching to maintain the stability of the dynamics. I’m all for going into space for many good reasons. We just have to be careful and mindful.
@Bill Christie
It’s not just discussed, but actively monitored!
The retro-reflectors left on the Moon by the Apollo missions still work and allow anyone on Earth (with a powerful laser and telescope) to bounce light off of them and use the round-trip time to calculate the distance to the Moon. The “Lunar Laser Ranging Experiment” is ongoing and has found, among other things, that the Moon is steadily moving away at about 4cm per year.
What’s the point of going to the Moon?
Great Explanation of physical laws-constraints concerning the moon
As a retired physicist and business executive I suggest you publish a few follow ups!
1 Why Special Relativity speed limit -the velocity of photons-prohibits any humans from reaching the stars
and by symmetry laws forbids aliens from reaching us
2 The RETURN ON INVESTMENT for developing moon colonies for private businesses is not justifiable which means taxpayer billions will be required but not justified when contrasted rerouting that money to earthly project like healthcare for all and cures for cancer
3
For the maglev/coil gun system if you where to angle it up using some of the mountains near the base would that not reduce the distance required for the track?
I play KSP and would love to have this option available to test out the theory of using this launch system.
I am concerned though over the amount of power (eV) required before using any propellent.
But would make trips to the rest of the solar system much faster. For example a coil gun launch and a propellent rocket the size of the shuttle could be getting to Mars within 3 months. This being due to acceleration and deceleration all the way.
OR is my maths wrong in this matter?
@Ian Bennett
Rather than a ramp, that would involve a sudden “ramping up” of g forces at the end of the ride, you can point the track where you like by finding the location on the Moon where the surface is lined up just right and building there.
Weirdly enough, when it comes to escaping bodies in space, your speed is important but your direction isn’t. If you’re moving at escape velocity in any direction (that doesn’t intersect the surface), then you’ll escape. Move slower in any direction and you don’t. So an adjustable ramp could help you aim, but it wouldn’t help get you get away from the Moon.
Yes I was thinking of the long distance ramp idea not the sudden “kick up” or “ski ramp” like on small aircraft carriers.
So if that is the case why launch rockets straight up? I would guess it is the air friction you want to overcome quickly and so higher mean less resistance.
I know it would not work for any small bodies, space stations etc., due to mass dV offsets but on the moon, large asteroids or other planetary moons it would work brilliantly.
Now if you able to keep the propellent from being used until after the coil gun had gotten you to orbit, or nearly there, then how much quicker would a trip to say Mars be?
I am thinking here of the dV required for lunar orbit for a large ship, say 200t, and that being saved for acceleration/deceleration during the trip
@Ian Bennett
Exactly right. When rockets leave Earth they start by moving up because the higher they are, the less they have to fight their way through air. When something moves at orbital velocity through the lower atmosphere, they burn up. That’s why we don’t worry about de-orbiting satellites (much).
The lowest-energy and most gentle route between planets is a Hohmann Transfer Orbit, so when you hear a particular delta V quoted that’s almost always what they’re taking about. You can get from place to place faster, but the faster you go the more the trip feels like being in a bullet, both at the beginning and end, and the more energy gets wasted.
I was thinking of the resupply missions from the movie “the Martian” they had alternative options than HTO.
Am thinking here of tourism. If you can get to Mars quickly, say maintaining .8g, the entire way, with turn over etc. It might be expensive but practical for people to do it as tourists
I wonder also if they had done the steel eyed Purnell maneuver as soon as they had know Mark was alive they would have had two supplies loaded and heaps more dV.
Could an ion engine be useful for “fast” interplanetary missions?
@Ian Bennett
I hadn’t realized what a nerd Andy Weir is. He actually bothered to model the orbits and trajectories accurately, including the positions of Earth and Mars in the 2030’s. This is an analysis of the orbits used in the book. It turns out they never quite use an HTO, because they use an ion drive to accelerate (slowly) the entire time, but the trip out is close. During the rescue they take a longer path, swinging closer to the Sun instead of staying between Earth and Mars’ orbits (which is what an HTO does). That rescue path takes a lot longer, in part because they’re not using an HTO.
I normally focus on “cheap and dumb” spacecraft (e.g., a hollow brick), because although ion drives are the most efficient way to get going fast, they have practically zero acceleration. If you were on a ship with a modern (expensive/delicate) ion drive, you’d only notice it was working because the things floating around the room would gradually accumulate on one wall. Constant 1g acceleration would be great, but it would be stunningly costly (energywise) and we’re probably centuries away from that happening, if it ever does.
hmm
And even if all these practical and legal challenges are overcome, what would it actually be like to live there? Frederic Marin, an astrophysicist at the University of Strasbourg in France, explains just how inhospitable it would be to live in an environment with such low gravity, covered in abrasive dust. And Rowena Christiansen, a medical educator and doctor at the University of Melbourne in Australia, talks through some of the side effects that spending prolonged amounts of time in space can have on the human body.